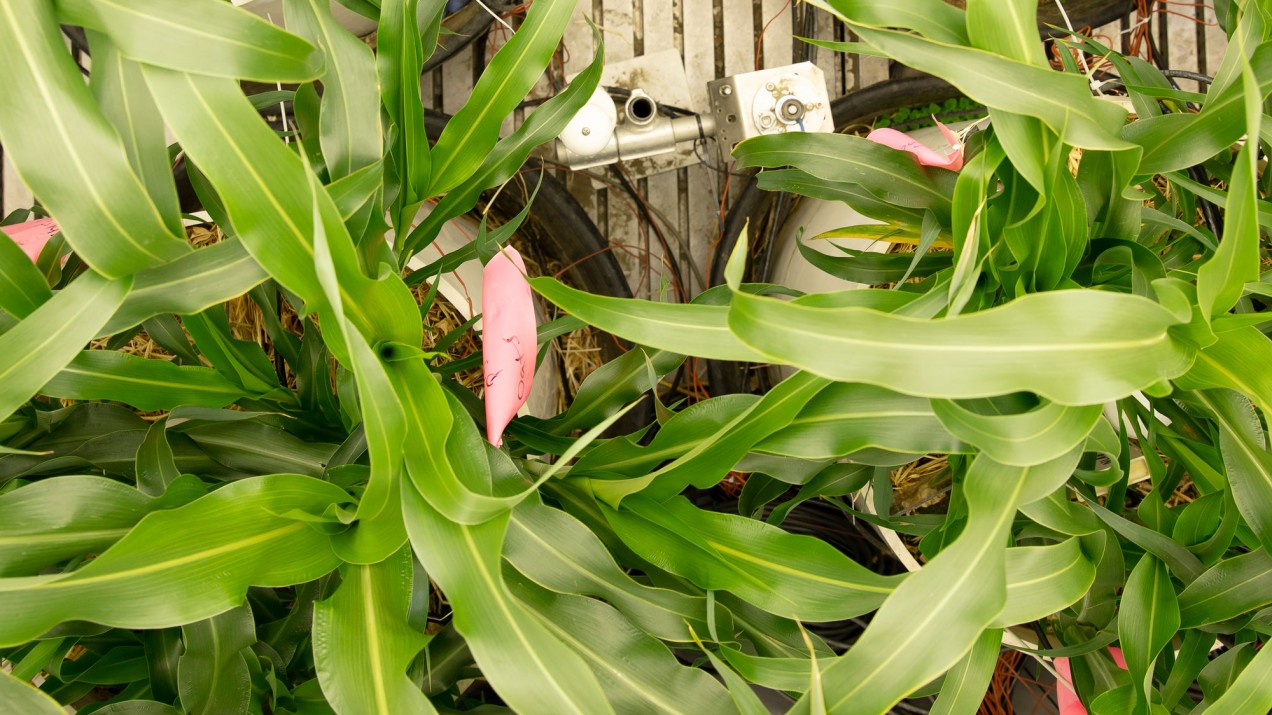
It’s a bitter cold March morning in Ames, Iowa, and the sprawling cornfields outside of town are buried beneath a couple of inches of ice and snow. But it’s hot and humid inside the custom-built grow chambers on the campus of Iowa State University.
Blindingly bright lights beat down on a trio of squares, each containing close to 7,000 pounds (3,175 kilograms) of soil, sunk five feet (1.5 meters) into the floor. The steady churning of fans, ensuring air circulation and uniform temperatures throughout the room, echoes off the walls. Every few inches, a suite of infrared thermometers and moisture sensors track the microclimates surrounding the leaves of the plants.
Inside these growth chambers, it’s the future. And Jerry Hatfield, an affable agronomist who heads the US Department of Agriculture’s National Laboratory for Agriculture and the Environment, doesn’t like what he sees.
Three years ago, Hatfield used the growth chambers to find out how local crops would perform under the temperatures predicted for the region in 2100, which are expected to rise roughly 4 °C on average, or about 7.2 °F. He simulated a growing season, from April 1 through October 30, for three different strains of corn used by farmers in the area. In one chamber, Hatfield started the temperature at just around 50 °F (10 °C) to mimic conditions in early April, raised it well above 100 °F (38 °C) to simulate the hot summer days (as high as 114 °F in the chamber with 2100 conditions), and then brought it back down again for autumn. In a second chamber, he simulated the region’s current, cooler climate norms.
The differences between the plants in the two chambers were stark. While the leaves looked the same, the impact of that extra 7.2 °F was far worse than projected by even the most pessimistic scientific literature. The number of corn kernels per plant plummeted, in some cases by 84%. Some plants produced no kernels at all.
It was just the first in a series of alarming results. In the months that followed, Hatfield and his colleagues simulated the rising temperatures and altered rainfall patterns expected to hit the wheat fields of Salina, Kansas, as soon as 2050. Yields fell as much as 30% with low precipitation and as much as 70% with the combination of high temperatures and low precipitation expected in the decades ahead.
To date, it’s been relatively easy for American farmers to shrug off climate change. After all, under the most optimistic models, projected US yields for corn and soybeans—which are grown on 75% of the arable land in the Midwest—are actually expected to increase through 2050, thanks to warmer weather that will benefit the relatively cool northern climes. But after that, if Hatfield is right, yields will fall off a cliff, devastating farmers and leaving much of the world hungrier.
By 2050, the world’s population is expected to grow to 9.7 billion. As living standards and diets also improve around the world, food production will have to increase by 50% at a time when climate change will help make both sub-Saharan African and East Asia unable to meet their own needs without imports. Already US corn and soybeans account for 17% of the world’s caloric output. The UN Food and Agriculture Organization projects that American exports of corn must almost triple by 2050 to meet the shortfall, while US soy exports would have to rise by more than 50%. All this extra food has to be grown without using significantly more land. That means it’s going to be all about yield—the productivity of the crop.
And that is what has Hatfield so worried. A growing body of scientific literature suggests that climate change is likely to decimate yields unless we can find new ways to help plants cope with the droughts, vast temperature fluctuations, and other extreme weather that’s likely to become commonplace in the decades ahead.
“If something isn’t done, we will see major drops in production across large areas of the corn belt and Great Plains,” Hatfield says. “Either we’re going to change the crops that we produce or we’re going to have to think about how we genetically manipulate that plant to have a higher tolerance to higher temperature.”
There is, of course, a familiar ring to the dire predictions. World leaders in the early 1970s were so worried that rising populations, increasing pollution, and soaring food prices would create an acute food crisis by the dawn of the 21st century that the UN convened a conference in Rome. “Time is short,” the member states declared after the conference, in 1975. “Urgent and sustained action is vital.”
In the years that followed, however, high-yield crops, a wider use of irrigation, farm mechanization, and the introduction of synthetic fertilizers and pesticides led to a “Green Revolution,” dramatically increasing agricultural production in many places around the globe.
Now the pace of growth has begun to slow. Water is short in many areas, limiting further expansion of irrigation. And it’s hard to imagine using even more fertilizers and pesticides. “It’s already an open question whether we will be able to keep inventing these new technologies and management practices that allow productivity to more than keep up with demand,” says Marshall Burke, a Stanford economist who focuses on climate change. “But climate is for sure going to make that a lot harder.”
What’s more, global warming is already making its effects felt. In 2011, Columbia University economist Wolfram Schlenker and Stanford ecologist David Lobell looked at what happened to crop yields as temperatures rose between 1980 and 2008. They found that global maize production (excluding the US) fell 3.8% and wheat production dropped 5.5% relative to what it would otherwise have been. The increase in hot days and nights explains about half of all variation in corn yield. Higher temperatures help up to a point, between roughly 50 °F and 84 °F, but hotter than that and yields plummet.
To get a sense of what this all might do to global food prices, Schlenker suggests looking at what happened in 2012, the last time the American Midwest experienced a summer with temperatures comparable to what climatologists project will become the norm by the end of the century. The region’s production of corn fell by 25% and soybeans by 10%. That constitutes about a 4% to 5% drop in total global caloric production—conditions under which we can expect food prices to spike by as much as 30%, he says.
Despite a few such bad years, however, Midwest farmers have enjoyed a decades-long rise in productivity, masking the worries about the future. There is a “Goldilocks” zone for temperature, humidity, and rainfall, and climate change has, for the most part, pushed the middle of the United States further into it, says Gene Takle, former longtime director of Iowa State’s climate science program. The largest change in Iowa to date has been increased rainfall in April, May, and June— it’s up by almost 25% over the last three decades. This extra rain, caused by the interaction of wind patterns with warming waters in the Gulf of Mexico, has forced farmers to spend more money on drainage tiles and the like to adapt, and it has shifted the planting season. But the combined result of technological advances and more favorable climate is that yields have risen 28% across the Midwest. “There’s pretty good agreement that climate change has been favorable to agriculture to this point,” Takle says.
These trends will reverse, though; where the experts disagree is on precisely when. Takle cites one model showing that the positive trend in productivity will turn around by 2035, undoing all the gains seen since 1981. And yields will only continue to fall from there. “We’re on the cusp right now,” he says.
On a recent day, he pushed a piece of paper across a table. It was full of colorful charts and bullet points, detailing the impact of climate change to date on local agriculture, with the positives spelled out in green and the negatives in red. Among the red points: more pests were surviving the winter, and waterlogged soil reduced the number of days farmers could work the fields. (In 2013, northwest Iowa had 700,000 acres—283,000 hectares—that couldn’t be planted for that reason.) But there was plenty of green, too.
Then he turned the page to show how things would look in 2050. There was no green on this page—only a long list of red. Intense spring rains will make field work early in the season harder, Takle expects. There will be more flooding; the warmest day will be 7 °F hotter. Every other year will see at least one five-day period when extreme heat will cause corn and soybean pollination to fail and vegetative growth to stall.
“We have a lot of problems coming down the pike,” he says.
The job of Hatfield, the USDA agronomist, is to monitor the impact of environmental conditions on the country’s farmers and identify potential solutions. Sitting in his office on a recent day, he ticked off a long list of worries. The brown marmorated stink bug appeared in the US in the late 1990s, and as temperatures rise over the next 30 years, its range will expand all the way up to Canada, damaging a wide array of crops. The Palmer amaranth, an herbicide-resistant weed that lives in particular microclimates and has so far threatened mainly soybean and cotton crops in the South, will likewise spread northward and become ubiquitous.
Then there’s the increasing soil salinity in North and South Dakota, which could take significant tracts of land out of rotation in less than a decade if current trends continue unabated. The problem is that thanks to the changing climate and changing financial incentives, farmers in the Dakotas who have traditionally grown wheat, sugar beets, hay, and canola in rotation are increasingly switching to corn and soybeans. But the new crops are poorly suited to the wet early springs of the Dakotas, allowing the water table to rise and evaporate, leaving behind salt that damages the soil.
The biggest worry is what climate change will do to crop yields in America’s breadbasket in the decades ahead. Hatfield has become convinced that no policy fix or change in management practices alone will be enough to overcome the natural limits of plants and the coming extremes of weather. Which is why he has decided to turn to advanced genetics.
In the coming months, Hatfield plans to repeat his experiment simulating future growing seasons. But this time he will examine precisely how the conditions affect which genes are turned on and off in corn and wheat. The hope is to identify ways to control these molecular-level switches to help the plants adjust to the conditions they will face.
It’s early days for the work on corn, wheat, and soybeans. But in other crops, breeders have identified adaptations and harnessed them to solve challenges posed by climate change. Some of the most successful have been seen in the rice-growing areas of the world.
Rice can tolerate a certain degree of flooding—can actually benefit, because the flooding can kill weeds. As long as the rice stalks are partly above water, the plants can absorb the air they need. But if the tops of the plants are submerged, they can suffocate.
“You want to eat in the future? That’s what’s at stake. But we’re going to have to figure it out, because we have no other choice.”
In 2006, researchers cloned a gene called Sub1 in a local South Asian rice strain. The gene confers “submergence tolerance” on rice. When the plant is underwater, the gene is turned on and puts the plant into suspended animation. The gene turns off as soon as the top of the rice shoot is back above water. Unlike some traits that increase stress tolerance, the Sub1 gene does not seem to significantly reduce yields when there are no floods—and when there are, the plant resumes growing with extra vigor afterwards, says Susan McCouch, a professor of plant breeding and genetics at Cornell University.
In 2009, the International Rice Research Institute engineered the trait into eight varieties of rice and began to distribute them to farmers. It is now used by 10 million farmers, on about 10 million hectares of land.
Though nothing comparable to the water resistance in rice has yet been found for corn, commercial seed companies have been breeding drought-resistant strains for more than a decade. Robert Reiter, head of R&D for Bayer’s crop science division, explains how the company searches for resilient breeds. First, it digitizes DNA sequence information and catalogues known traits for several million lines of plants. Using the data, it then trains machine-learning algorithms to screen millions of other strains for useful traits.
For instance, Bayer has bred traits picked up from heat-tolerant and drought-resistant strains in Mexico into high-yielding strains adapted to the US market. Reiter says that during a 2012 drought in the US Midwest, yields were more than double what they were in 1988, the last time a drought of comparable severity struck.
But the approach has its limits. “Plants can only tolerate a certain level of stress, and timing is a big factor,” he says. “If you have extremely high temperatures during the process of flowering, you will see some impact on the yield … What we can do is just try to minimize the impact.”
Hatfield hopes to overcome some of these limitations by simulating future growing conditions. Such simulations, he hopes, could help identify unknown genetic pathways that plants might use to adapt to future changes in weather and growing conditions.
It’s a complex puzzle that we’ve only begun to work on. And Hatfield notes that it’s difficult to account for the combination of factors Midwestern farmers are likely to face, including changing precipitation patterns, extreme heat, dramatic swings in weather, a whole host of new pests, and perhaps challenges we have not yet even anticipated. But he’s clear about why he’s doing it.
“You want to eat in the future?” he says. “That’s what’s at stake. But we’re going to have to figure it out, because we have no other choice.”