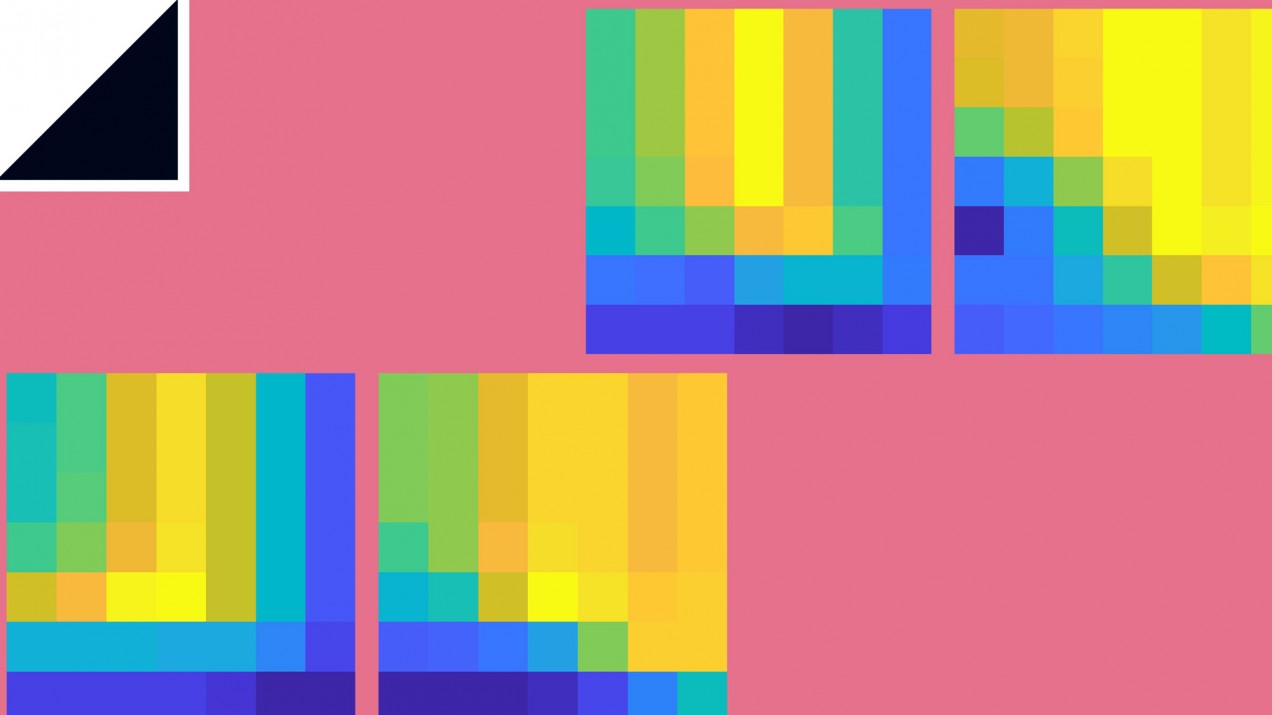
At almost every wavelength, engineers have electromagnetic antennae that can detect and record the waves and create exotic images of the world at radio, microwave, infrared, visible, and x-ray frequencies.
But there is a blind spot in this spectrum. The technology is still in its infancy to detect radiation with a wavelength of between 1 and 0.3 millimeters and a frequency of about a terahertz. The equipment that can detect such radiation is bulky and expensive and the resulting images poor. Hence the “blind spot,” which engineers have called the terahertz gap.
A better way to capture these wavelengths is desperately needed, not least to gain a new window into the universe.
Today Martin Burger at the University of Munster in Germany and a few colleagues describe a revolutionary new imaging technique—compressed sensing—that is set to make this part of the electromagnetic spectrum more accessible. Applying the technique to terahertz waves is likely to change the way we see our world and the universe beyond.
First, some background. Terahertz waves pass through clothes but not through skin or metal. If your eyes could pick them up, people would appear naked but decorated with keys and coins but perhaps also knives and guns. So this kind of imaging has significant security applications, not to mention privacy implications.
Terahertz frequencies are difficult to detect because they sit on the electromagnetic spectrum between microwaves and infrared light, and there is an important difference between the way these types of radiation can be detected.
Microwaves, like radio waves, are made by accelerating a charge back and forth at the required frequency—in this case, up to about 300 gigahertz. Detecting microwaves exploits the same process in reverse.
By contrast, infrared waves, like light, are made by making an electron in a suitable material jump between two electronic levels. This generates infrared light when the energy required to make the jump is equivalent to the energy of an infrared photon. The same process in reverse can also detect infrared photons.
Making and detecting terahertz waves is hard because they sit in the middle where neither technique works particularly well. It's tough to accelerate charges at terahertz frequencies. And materials with the required bandgap to create terahertz photons are difficult to find, and those that qualify often have to be cooled to cryogenic temperatures. That’s why terahertz detectors tend to be bulky, expensive, and hard to manage.
But compressed sensing can help, say Burger and co. In recent years, this technique has taken the world of imaging by storm because it allows a single pixel to record high-resolution images, even in 3-D.
The technique works by randomizing the reflected light from a scene and then recording it using a single pixel. The randomization can be done in various ways, but a common approach is to pass the light through a digital array called a spatial light modulator that displays a random pattern of transparent and opaque pixels. The randomization process is then repeated and the light field recorded again, and the entire process is repeated many times to generate many data points.
At first it’s hard to see how this can produce an image—after all, the light field is randomized. But the data points aren’t completely random. Indeed, each data point is correlated with all others because they all come from the same source—the original scene. So by finding this correlation, it is possible to recreate the original image.
It turns out that computer scientists have a variety of algorithms that can do this kind of number crunching. And the result is an image with a resolution that depends on the number of data points recorded by the pixel. The more data, the higher the resolution.
That has immediate application for terahertz imaging. Until now, the only way to create a 2-D image was to use an array of terahertz detectors or to scan a single detector back and forth to map out the light field. Neither technique is satisfactory because of the unwieldy size of terahertz detectors.
But compressed sensing offers an alternative: using a single terahertz detector to record multiple data points through a spatial light modulator that randomizes the terahertz light. That works well for visible and infrared light, and numerous groups have begun to exploit it successfully.
However, terahertz light introduces some additional complexities. For example, because terahertz waves are two or three orders of magnitude bigger than optical waves, they more easily diffract. This effect and others introduce distortions that make the image reconstruction much harder. It is this challenge of image reconstruction that Burger and co have taken on.
Their results are impressive. The team shows how various techniques can significantly improve the quality of resulting images. “The compressed-sensing approach based on single-pixel imaging has great potential to decrease measurement time and effort in THz imaging,” they say.
However, there are challenges ahead. One problem is in dealing with images made from more than one frequency of terahertz light. This kind of analysis is particularly important because it provides spectroscopic information about the chemical makeup of the subject in the image—for example, whether a crystalline powder is flour or some kind of drug.
But this requires different types of mask. So a challenge is to find the best way to create a hyperspectral image using the smallest number of masks.
Nonetheless, Burger and co are optimistic that compressed sensing will allow rapid progress in finally closing the terahertz gap.
Ref: arxiv.org/abs/1903.08893 : Reconstruction Methods in THz Single-Pixel Imaging