Engineering microbial worlds
A family loss intensified Cullen Buie’s interest in bacteria—and harnessing them for good.
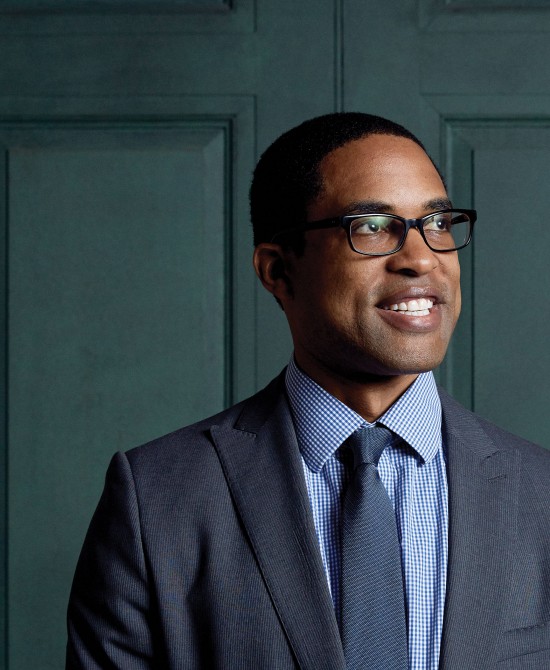
Of the world’s myriad species of bacteria (estimates range from millions to billions, depending on who you ask), scientists have identified and catalogued only some 20,000. And among those species, fewer than 1 percent have been subjected to the kind of tinkering that could help researchers in the booming field of genetic engineering adapt them for countless human purposes.
“There’s just so much we don’t know,” says Cullen Buie, the recently tenured Esther and Harold E. Edgerton Career Development Professor of mechanical engineering. “All of the work is being done on 1 percent of 1 percent. We’re literally just scratching the surface.”
But the potential is enormous: by altering the genetic code of existing bacteria, researchers in the not-so-distant future may be able to build microorganisms that can do things like treat diseases, create fertilizer on demand, or clean up oil spills.
“There’s all this motivation to try to harness the microbial world, and people are realizing, ‘Man, we’re limited by our tools,’” he says. So Buie is using his microfluidics expertise to create devices that he hopes can “really shed light on all kinds of problems that they just couldn’t even look at before.” At MIT, he directs the Laboratory for Energy and Microsystems Innovation (LEMI), which focuses on electrochemistry, microfluidics, and fuel cell research, much of it aimed at finding new ways to manipulate bacteria. He has also cofounded a startup developing a microfluidic tool that could dramatically accelerate the pace of genetic engineering.
Buie didn’t initially set out to study bacteria. As a teenager, he’d intended to pursue medicine, following in the footsteps of his older sister, Simone. But attending a pre-college engineering camp led him to major in mechanical engineering at Ohio State. Then, as a National Science Foundation graduate research fellow at Stanford, he found himself drawn to renewable energy—and to micro- and nanotechnologies. So he joined the lab of mechanical engineering professor Juan Santiago, who had just received funding for a project building nanoscale pumps for vehicle fuel cells. “I was like, ‘Wow, this is perfect. This is alternative energy and microscales all at once,’” he says.
Under Santiago, Buie focused on microfluidic “electro-osmotic” pumps that use electrical fields to regulate pressure and flow rate. For his dissertation, he explored their use in low-temperature fuel cells. In a postdoctoral fellowship at the University of California, Berkeley, Buie turned his attention to microbial fuel cells, which are often used in biosensors and wastewater treatment facilities. Microbial fuel cells contain bacteria attached to an electrode. When given organic matter from sources like wastewater, the bacteria break down the food, allowing electrons to be harnessed to create an electric current.
The following year, bacterial work became personal. In 2010, shortly after he’d joined the MIT faculty as an assistant professor of mechanical engineering, he got a phone call. His sister -Simone had been admitted to a hospital in Ohio earlier that day with a raging fever, rapid heartbeat, and pain. She died hours later of sepsis, a condition that occurs when the body’s efforts to fight off common bacteria like Staphylococcus aureus (staph) and Streptococci trigger inflammation leading to organ failure.
Buie was devastated. As he worked through his grief, he started thinking about his sister’s death from a scientific perspective. “It was a little bit shocking to me that people still died of bacterial infections,” he says. “I was already working on microbial fuel cells, and so when this happened, it piqued my curiosity around bacteria and all the different things they can do, both good and bad.”
At the time, LEMI was just getting off the ground, and its first students and postdocs were headed down disparate research paths. One project focused on new ways to fabricate superhydrophilic (water-absorbing) and superhydrophobic (water-repelling) surfaces—techniques that could be useful in naval engineering—while another centered on designing a new type of battery that uses fluid dynamics to keep its components separate.
Meanwhile, other LEMI researchers focused on developing microfluidic tools that would use a process called dielectrophoresis to sort bacteria according to their cells’ electrical properties, which include polarization, the buildup of charge on their surface. For this project, Buie’s team used microfluidic channels the width of about five human hairs, with bottleneck points about one-tenth that size in the middle. They pushed cells of Pseudomonas aeruginosa bacteria through the channels and applied electrical fields. As they increased the voltage, different bacterial strains glided through the channel while others stopped at the bottleneck, where the electrical field was most intense.
Precisely where a cell stopped—and how intense the electrical field was at that point—showed researchers how polarized the surface of the bacterium was, providing clues about its pathogenicity. More pathogenic strains of Pseudomonas aeruginosa are more likely to polarize at lower voltages, which led Buie to think that the research could be applied to help diagnose bacterial conditions like sepsis in time to save lives.
More practical methods already existed to determine whether bacteria are pathogenic, however. Now, Buie is focusing on using dielectrophoresis for connecting genetic information to the physical properties of bacteria. If, for example, genes are knocked out of a little-studied bacterial strain, any resulting changes in polarization can offer “some information on what might be the utility of those genes or what area of the cell they might be affecting,” he says.
By 2013, Buie had turned his attention to finding a way to dramatically speed up genetic engineering. Researchers had long been inserting different kinds of DNA into cells to try to get them to, say, fight pathogens or metabolize carbon dioxide to help mitigate climate change. But tools for delivering the foreign DNA were not advancing as fast as the strategies for harnessing the manipulated cells, he learned after meeting a representative of the Defense Advanced Research Projects Agency at a talk about synthetic biology.
To get DNA into cells, many researchers rely on electroporation—a method of using finely tuned electrical pulses to temporarily open pores in cell membranes. But the process requires researchers to know the exact electrical field that will open the pores without killing the cell. Finding that specific field, and the right growth medium for any given bacterial strain, can take years. And once that’s done, the process of preparing, pipetting, and electrically zapping each sample is painstakingly slow. Buie estimates that one skilled researcher can electroporate only 20 to 50 samples per hour, significantly restricting the number of experiments a lab can conduct.
So with the help of a DARPA grant, Buie began working on a faster way to electroporate cells—and eventually, he would also tackle automating the process. His team—which included LEMI postdocs Paulo Garcia and Jeffrey Moran as well as graduate student Zhifei Ge, PhD ’16—used a setup similar to the one in Buie’s previous dielectrophoresis experiments, but they added to the microfluidic channel a fluorescent marker that would glow in the presence of DNA. Channels were filled with bacteria, and as electrical fields increased around the bottleneck, membrane pores opened, letting in the marker. Once inside, it reacted with the bacteria’s DNA and made the cell glow, providing a visible indicator of the electrical field needed to open a particular strain’s pores.
Once researchers know that, they still face the laborious task of manually zapping each cell to insert the desired DNA. So Buie, -Garcia, and LEMI graduate research assistant Rameech -McCormack, SM ’17, also designed a pipette that applies the correct electrical field as the cells flow through electrically charged microfluidic channels built into its tip. Through their startup, called Kytopen, Buie and Garcia (who serves as Kytopen’s CEO) are engineering an automated system equipped with 96 or more simultaneously electroporating pipettes, each of which can tackle one sample every eight to 10 seconds. By making it possible to insert DNA into bacterial cells up to 10,000 times faster, the device could allow researchers to rapidly churn through millions of variations on an experiment in genetic engineering. (Kytopen is among the first companies supported by The Engine, MIT’s venture fund/accelerator for early-stage startups working on technologies with big potential but long development time lines. See “Investing in Tech That’s Worth the Wait,” March/April 2018.)
Buie’s next step is to test-drive his electroporation device on organisms that scientists aren’t yet able to genetically engineer, and he’s starting with bacteria in the mouth. The Forsyth Institute, a biotechnology research nonprofit, has isolated hundreds of varieties of human oral bacteria. Buie has teamed up with Forsyth’s Christopher Johnston, a microbiology researcher who’s developing methods of dodging cell defense systems that reject foreign DNA. Together, they aim to make at least 200 bacterial strains available for genetic engineering.
If they succeed at significantly expanding the number of organisms scientists can manipulate, the research could one day be used to engineer bacteria for important applications in health care, energy, agriculture, and environmental science.
Buie hopes his work will empower other researchers to take on more difficult questions. “People will stop saying ‘I can’t work on that bug’ because they can’t do genetics,” he says. “They’ll say, ‘Why don’t we try this?’”