Sustainable Energy
The race to invent the artificial leaf
In this excerpt from his new book Taming the Sun, Varun Sivaram follows the research paths of two rival scientists determined to find a way to wring fuel out of thin air.
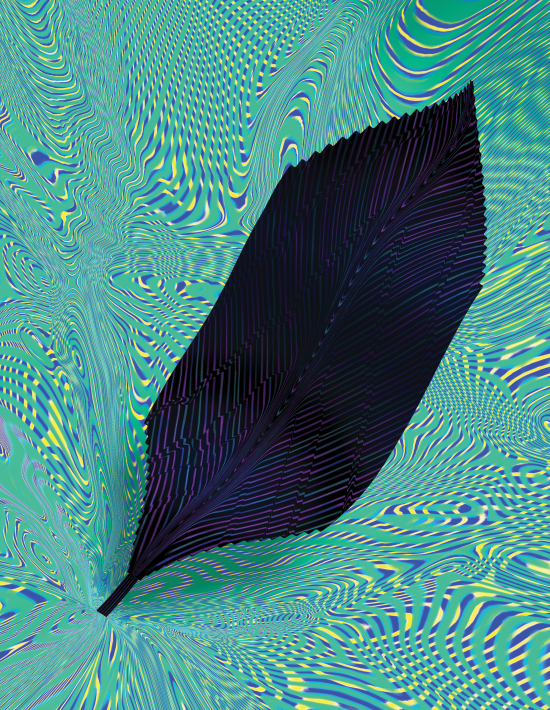
Since the early 1970s, scientists have been on a quest to develop a technology that could create liquid fuels out of carbon dioxide, water, and sunlight far more efficiently than photosynthesis, the process by which plants harness sunlight to produce carbohydrates and store energy. They call it the artificial leaf.
A commercially viable artificial leaf would solve several of the trickiest challenges in clean energy. It would create a way to directly and affordably store solar energy while producing a carbon-neutral fuel that could transform the transportation sector, even offering a way to make long-distance air travel environmentally sustainable.
Scientists have made slow but considerable progress on the two crucial steps in the process: developing catalysts that use solar energy to split water into oxygen and hydrogen, and creating others that can convert hydrogen and carbon dioxide into an energy-dense fuel. The remaining trick is to combine these tasks in an affordable and scalable way, using cheap and abundant materials.
In the following excerpt from his new book, Varun Sivaram, a physicist and fellow at the Council on Foreign Relations, explores the recent progress and diverging research paths of two rival scientists determined to finally deliver and commercialize the artificial leaf: Nathan Lewis at Caltech and Daniel Nocera at Harvard University.
On a balmy Beverly Hills evening recently, members of the Council on Foreign Relations gathered at the Peninsula Hotel to listen to a scientist share his vision for creating an artificial leaf.
Among the collection of executives and ex-ambassadors, most were unsure what to expect. A few exchanged nervous glances as I introduced the speaker’s credentials, perhaps bracing for an abstruse physics lecture.
But my guest that night, Nate Lewis, a professor at the California Institute of Technology (Caltech), is a rarity among scientists for his ability to condense complex concepts into memorable soundbites and weave his assorted research strands into a compelling narrative. Lewis, whose gray hair is testament to the decades he’s spent chasing an artificial leaf, kicked off his remarks on the future of solar power with this pithy refrain: “Can’t store? No power after four.”
That failing, he argued in his low, drawling voice, means we urgently need to develop technologies able to store the sun’s energy in a fuel that can be used when needed. His preferred route, an integrated solar fuel generator, is an elegant device that takes in water and sunlight and spits out gaseous hydrogen and oxygen. That hydrogen can then be used to fuel vehicles, generate electricity for the grid, or serve as a feedstock to make more complex fuels such as gasoline.
Lewis, also a principal investigator at the federally funded Joint Center for Artificial Photosynthesis, wants his artificial leaf to outperform nature’s best plants. Plants, for all their success, are actually terrible at converting sunlight into energy. Even if you don’t know anything about how photosynthesis works, you can tell from the leaves’ green color that totally efficient energy conversion might not be a plant’s top priority (black leaves would be much better at absorbing the sun’s rays). The green chloroplasts in leaf cells function well enough for a plant’s needs. They perform complex chemical reactions that, fueled by the sun’s energy, turn carbon dioxide and water into the energy-storing sugars needed for such activities as surviving and reproducing. When all is said and done, the most efficient plants convert barely 1 percent of the incoming sunlight into stored energy.
Vegetation nonetheless offers a generic model for turning sunlight into fuel. Early on in photosynthesis, plants split water and generate hydrogen and oxygen. The oxygen goes into the atmosphere, while the hydrogen feeds into subsequent chemical reactions.
The way plants accomplish this water-splitting is instructive. The first lesson is that they separate the two halves of the water-splitting chemical reaction—that is, the “half-reactions” that produce hydrogen and oxygen. Evolution was no pyromaniac, and this design choice prevents hydrogen from spontaneously combusting in the presence of oxygen. Second, the plant contains catalysts, or molecules that speed up the half-reactions. Third, plants separate the two half-reactions with a membrane that not only keeps hydrogen and oxygen apart, but also allows charged ions to pass through it, which is important to avoid an imbalance of charge.
Researchers developing solar fuel generators likewise need to put together a similar set of components. Two materials known as “photoelectrodes” are immersed in water and absorb light energy to perform each of the two half-reactions to split water. Two catalysts speed up each of those half-reactions. And a membrane stops the whole contraption—called a “photoelectrochemical cell” (PEC)—from exploding.
But the similarities end there. As Lewis likes to say, after taking inspiration from feathered birds, humans ditched the feathers and invented the 747. Unlike plants, the solar fuel generators of the future probably will not use two green photoelectrodes that compete with each other to absorb the same part of the sun’s spectrum. Rather, one of them—the anode, which creates oxygen from water—should harness colors of light toward the blue end of the spectrum, and let the colors toward the red end of the spectrum pass through to be absorbed by the cathode below, which produces hydrogen.
Producing affordable energy will require extremely cheap and abundant materials. But that’s not all the PEC has to do. To succeed it really needs to be not only cheap, but also safe, robust, and efficient. Unfortunately, so far researchers have only managed to create devices with no more than three of those four characteristics.
Start with safety. To prevent hydrogen and oxygen from combining and exploding, a PEC needs a membrane that separates the two half-reactions. But the half-reaction that produces oxygen from water also turns that water acidic, whereas the half-reaction that produces hydrogen turns nearby water basic. Scientists have to find materials for photoelectrodes and catalysts that do not get dissolved or corroded in acidic or basic media. That demand rules out many cheap materials that wouldn’t survive under such conditions. Therefore, making a solar fuel generator out of cheap materials and equipping it with a membrane to ensure safety can lead to it failing the robustness test.
Next, consider the amount of the sun’s energy that the device converts into energy stored as hydrogen. That efficiency depends on how well the photoelectrodes collectively absorb sunlight and how fast the two half-reactions split water. With carefully chosen photoelectrodes and catalysts, a solar fuel generator can theoretically achieve more than 30 percent efficiency. Expensive semiconductors offer a diverse buffet of materials to choose from, but cheaper compounds present a far more limited menu. Similarly, precious metal catalysts such as platinum are great at speeding up reactions, but they are rare and costly. The interdisciplinary team of researchers that Lewis led proceeded to throw massive computational power at the problem of finding materials that could satisfy all four criteria, systematically simulating thousands of compounds and testing out the most promising candidates in the lab.
Good old scientific intuition also played an important role in the research process—as has a little luck. Two examples stand out. First, Lewis and his collaborators found inspiration in the catalysts used in oil refineries to strip the air-polluting sulfur out of petroleum products. These catalysts are cheap, and excel at speeding up the half-reaction that produces hydrogen. (Unfortunately, researchers are still looking for a cheap, effective catalyst for the oxygen-producing half-reaction.)
Second, researchers in Lewis’ lab accidentally coated their samples with a thin layer of titanium dioxide and found a surprising result. Titanium dioxide is the key ingredient in sunscreen, which protects your skin by blocking ultraviolet rays of sunlight. Here, though, the ultrathin coating played a totally different role, protecting the photoelectrodes and catalysts from being eaten away by the basic solution.
Together, the borrowed insight from the oil industry and the accidental sunscreen discovery allowed Lewis and fellow researchers at Caltech to make a breakthrough. In 2015, they announced an integrated solar fuel generator that was over 10 percent efficient at converting sunlight into hydrogen fuel. The efficiency itself wasn’t any great leap—others had reached 22 percent efficiency. But the Caltech device used cheap, Earth-abundant catalysts, and it was able to pump out hydrogen over two days of continuous operation. As a proof of concept, it teased the possibility of a commercially viable product down the road.
If and when this technology leads to a commercial product, it is unlikely to look anything like the leaves that inspired it. Lewis envisions a tarp, rolled out across a vast expanse to soak up the sun’s rays, with drainpipes to collect the hydrogen that it produces. That is a far cry from the one-cubic-centimeter prototype his team created, but, listening to Lewis’ vision, it’s hard not to dream big.
Across the country from Nate Lewis, another acclaimed scientist is also on a quest to commercialize an artificial leaf. Like Lewis, Dan Nocera at Harvard University deftly combines science and communication, moonlighting as a science celebrity—something of a Carl Sagan for solar fuels. He has a knack for connecting with diverse audiences, from American Physical Society scientific gatherings to the Aspen Institute’s hobnobbing summits. If his crowd is dining on steak, he’ll warm them up by asking: “What did you just chew? The sun! The beef was just the energy of sunlight.”
Though both Lewis and Nocera share gray hair, the ability to engage broad audiences, and the same supervisor when they were in graduate school, their approaches to realizing an artificial leaf are dramatically different, resulting in a spirited professional rivalry. Whereas Lewis is laser-focused on producing hydrogen, Nocera wants to leapfrog hydrogen and build a device that harnesses sunlight to directly produce convenient, carbon-containing fuels that can immediately replace today’s petroleum products.
For a time, Nocera was content to focus just on hydrogen production. In 2011, he seized the scientific world’s attention by plopping what looked like a dark postage stamp into a glass of water, causing hydrogen and oxygen to bubble up on either side. Despite its simplicity, his artificial leaf was the culmination of 30 years of research, reaching back to his days as a graduate student at Caltech. Having made the breakthrough, Nocera set out to bring his new technology to market.
Unfortunately, he was about to learn the lesson that almost every other Silicon Valley clean energy startup has learned: the really hard part comes after making an exciting lab discovery. He would later lament, “I did a holy grail of science. Great! That doesn’t mean I did a holy grail of technology. And that’s what scientists and professors don’t get.” His startup, Sun Catalytix, ended up pivoting away from solar fuel to develop batteries to store energy for the power grid instead (Lockheed bought the company for an undisclosed amount in 2014).
But the experience hasn’t stopped him from chasing holy grails, so now, Nocera is pursuing the even harder target of harnessing sunlight, water, and carbon dioxide to produce carbon-based liquid fuels. The prospective benefits of such a technology are compelling. Liquid fuels already have enormous global infrastructure networks, including storage facilities, transcontinental pipelines, and oil supertankers, not to mention ubiquitous filling stations around the world. A device that could transform sunlight into fuels that are already commonly used could piggyback on that infrastructure.
Lewis maintains that the most promising route for making carbon-based fuels from sunlight involves solar-generated hydrogen as an intermediary. From there, well-understood industrial processes could combine hydrogen with carbon dioxide—captured from factories and power plants that burn fossil fuels—to produce a range of useful fuels known as hydrocarbons. A “solar refinery” could create the same range of hydrocarbon fuels that are produced today in oil refineries, and then employed as transport fuels or converted into a range of products from plastics to pharmaceuticals.
As futuristic as all of that sounds, Nocera wants to do something even harder. He wants to bypass the intermediate production of hydrogen and use sunlight, water, and carbon dioxide to directly produce carbon-containing fuels. If this maneuver could be done cost-effectively and at scale, it would be the most efficient, single-shot method of storing sunlight in the most versatile fuels known to humankind.
From a scientific point of view, this task looks nearly impossible. Just splitting water to generate hydrogen and oxygen is hard enough. But to create the simplest hydrocarbon—single-carbon methane, which makes up natural gas—is a far more complex proposition. That will require the discovery of even more new materials to absorb light and catalyze chemical reactions. As a result, a commercial technology to make carbon-based fuels directly from solar energy is much further away than one that can produce hydrogen.
Nevertheless, over the last three years, Nocera has made a string of improbable breakthroughs. The first was a conceptual shift: instead of using manmade devices to beat photosynthesis, why not harness nature instead? Nocera knew that nature uses intricate enzymes as catalysts in photosynthesis to convert sunlight into complex sugars. He realized that genetically engineered bacteria could behave similarly after being equipped with an arsenal of potent enzymes.
So in 2015, Nocera built a hybrid device that first split water using an inorganic catalyst to make hydrogen, as other artificial leaf technologies do. The same device then fed the hydrogen, along with pure carbon dioxide, to bacteria, which produced liquid fuels. But although the bugs were terrific at converting carbon dioxide and hydrogen into a variety of fuels, they were incompatible with the inorganic catalyst, which produced forms of reactive oxygen that destroyed the bacteria’s DNA.
Then in 2016, Nocera and colleagues published a paper in the journal Science triumphantly announcing a new catalyst, made from a cobalt-phosphorus alloy. It not only left the bacteria unharmed, but also self-assembled out of solution, mimicking the self-healing catalysts found in nature. With the catalyst and bacteria working together in harmony, Nocera’s device was able to achieve 10 percent efficiency in converting sunlight into alcohol fuels. Nocera reported that the bugs should be able to produce several other carbon-containing molecules for a range of applications from fueling vehicles to producing plastics. And he followed this up by demonstrating in 2017 that a hybrid catalyst-plus-bacteria approach could fix nitrogen in the atmosphere to produce ammonia. That is a tantalizing discovery because over 1 percent of global energy is used today in the production of ammonia to fertilize crops and feed the world. Nocera’s prototype suggests that one day, sunlight could power that process rather than fossil fuels.
The jury is still out on whether Nocera’s decision to harness living organisms is a good idea. Indeed, bacteria are quite finicky, sensitive to the acidity and temperature of their environment, and thus tough to design around. Smart money, for now, is on devices that harness sunlight to produce hydrogen advancing faster than those that try to directly produce carbon-based fuels. But, by combining modern materials with nature’s wizardry, researchers may yet leapfrog simple hydrogen in pursuit of a viable route to the ultimate holy grail: 100 percent clean, drop-in replacements for fossil fuels.
Excerpted from Taming the Sun: Innovations to Harness Solar Energy and Power the Planet by Varun Sivaram, published by the MIT Press. © 2018 Massachusetts Institute of Technology. All rights reserved.