Rewriting Life
Medicine's New Toolbox
An alternative way to make stem cells could open a window on human disease.
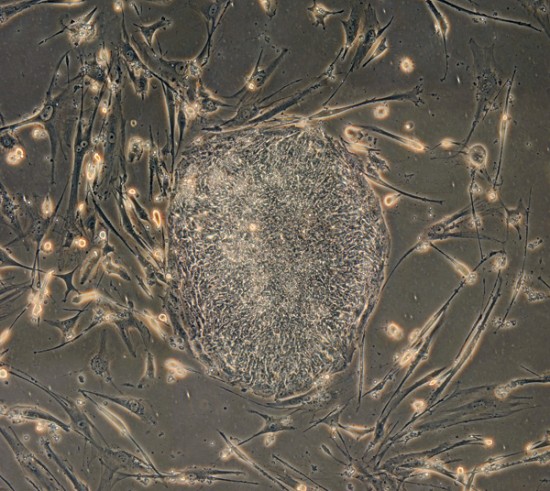
On the second floor of a building in one of South San Francisco’s numerous business parks, a new biotech company has set up shop. The walls sport a fresh coat of white paint, and the bench tops are shiny and bare. The tile floors are still glossy, and an expensive new cell-sorting machine sits, untouched, on the loading dock downstairs.
The building’s new inhabitant, iZumi Bio, is pursuing a technology as new and full of promise as the lab itself–a technology that’s moving faster than the company can fill its empty space. It revolves around induced pluripotent stem (iPS) cells: adult cells genetically reprogrammed to act like embryonic stem cells, which can turn into just about any type of cell in the human body.
Scientists have been talking about the medical promise of stem cells for more than a decade, even before human embryonic stem cells were successfully isolated in 1998. Most of the public attention has focused on their regenerative power: since stem cells can renew themselves and differentiate into specialized cell types, they could potentially be used to build replacement organs, heal spinal-cord injuries, or repair damaged brain tissue. But the research world has also pursued another, even broader-reaching goal: using the cells of patients with various illnesses to derive pluripotent stem cells, which can give rise not just to the specialized cells in a particular organ or tissue but to virtually any cell type. Those cells could be used to create laboratory models of disease. For example, a cell from a Parkinson’s patient could be turned into a neuron, which would exhibit the progressive molecular changes at work in the neurodegenerative disorder. This type of tool could capture the details of human disease with unprecedented accuracy, and it could revolutionize the way researchers search for new treatments.
Studying human disease in the lab is an enormously challenging task. It’s difficult to obtain brain tissue from a living Alzheimer’s patient, for example, and impossible to study how that tissue changes as the disease progresses. Animal models can offer only rough approximations of a human illness, capturing at best a few of its symptoms or causes. But iPS cells could yield a much more comprehensive picture. Because each cell line comes from a human patient, the cells reflect the complex array of factors that led to the patient’s disease: the genetic mutations, the effects of environmental history. And because those cells can be prodded to develop into a variety of tissue types, scientists can watch the disease unfold in a petri dish. They can observe, for example, the subtle molecular changes that take place in the neurons of a patient with Alzheimer’s long before the telltale signs of the disease, such as amyloid plaques, can be seen in the brain. It’s the difference between trying to piece together the details of a plane crash from photos of the wreckage and watching a video of the crash from every angle, with the ability to stop, zoom in, and rewind at will.
“The past two years have been nothing short of a revolution,” says John Dimos, a senior scientist at iZumi. “These cells didn’t really exist two years ago. This is all brand-new technology, and it’s opening up the potential for brand-new science.” The company plans to take advantage of that potential by developing a bank of iPS cells from patients with various diseases and using the cells to screen candidates for drug development.
Thousands of other labs are jumping at the chance to use iPS cells as well–whether to create new disease models, to study tissue development, or even to figure out how to build tissue for transplantation. Biologists say the field is charged with a kind of energy not seen since soon after the structure of DNA was discovered. “This is a really rare phenomenon in the biological research community,” says Sheng Ding, a chemist at the Scripps Research Institute in La Jolla, CA. “It’s a sensation, really. Everyone, more or less, is working on using iPS-cell technology for their specific research interest.”
Stem Cells 2.0
Scientists have been searching for ways to directly reprogram adult cells for decades. That hunt has been pushed forward by the desire to develop an alternative to human embryonic stem cells, which are fraught with both technical and ethical issues. The cells are usually derived from four- or five-day-old embryos that would otherwise be discarded from in vitro fertilization clinics (although sometimes embryos have been created expressly for research purposes). Using this technique to create a robust cell line is tricky and highly inefficient. Not only are the embryos themselves hard to obtain, but the cells are delicate and difficult to grow.
Another technique, human therapeutic cloning, is even more controversial, and both technically and practically challenging. Scientists transfer the nucleus of an adult cell into the hollowed-out shell of an unfertilized egg cell–which can then develop into an embryo, yielding stem cells that are genetic clones of the adult cells. But the lack of human eggs for research has proved a huge hurdle, and scientists have yet to generate cloned human cell lines.
But three years ago Shinya Yamanaka, of Kyoto University in Japan, figured out how to return adult mouse cells to an embryonic-like state in a process that never involved an actual embryo. He found that using a virus to deliver genes for just four specific proteins to the nucleus of an adult cell could give it the ability to differentiate into a wide variety of cell types, just like the stem cells derived from embryos. Those proteins, typically found in developing embryos, appear to turn other genes on and off in a pattern characteristic of embryonic rather than adult cells. A year after Yamanaka’s discovery, his group and two others reported that they could induce human cells to do the same thing.
As a physician and venture capitalist closely following stem-cell research, Beth Seidenberg saw the potential almost immediately. Seidenberg, a partner at Kleiner Perkins Caufield and Byers, teamed up with another venture capital firm, Highland Capital Partners, to found iZumi in 2007, funding the company with $20 million. After 20 years in pharmaceutical research, Seidenberg has had a lot of time to think about what the industry is doing right and where it’s going wrong. She says, “I became really intrigued by the idea of starting with a patient who had a disease and working backwards, which is exactly the opposite of how we pursue new therapies for treatment of disease today.”
To illustrate the role iPS cells could play in drug discovery, John Dimos points to amyotrophic lateral sclerosis (ALS), a neurodegenerative disease he has studied for years. About 2 percent of all cases have a known genetic cause–a mutation in a gene called SOD1. Nearly all the work in animal models has focused on this rare form of the disease, because researchers know how to use the gene to trigger it in mice. With the new technology, however, scientists can use a skin biopsy to generate pluripotent stem cells from any patient with ALS. The genetics and other possible factors underlying the disease are captured in the cells, even if no one knows explicitly what those factors are. The same holds true for Alzheimer’s, diabetes, autism, heart disease, and myriad other conditions whose complex origins have proved difficult to identify.
As a postdoc at Harvard, Dimos built a cellular model of ALS, making it possible to study a neurodegenerative disease outside an animal for the first time. He and his colleagues collected skin cells from an 82-year-old woman with ALS, reprogrammed them into iPS cells, and directed the cells to differentiate into motor neurons that were genetically identical to the donor’s defective cells. “It was the first paper to show that you can use a stem cell to see disease pathology in a petri dish,” says Douglas Melton, codirector of the Harvard Stem Cell Institute. “That means you can now study diseases in petri dishes and not in people. That’s huge.”
Because they are derived from human patients with documented medical histories, iPS cells are accompanied by reams of previously inaccessible information. “You can see from their medical history the progression of the disease, how they responded to different drugs, exactly what symptoms they experienced, and when,” says Dimos. Certain drugs may be more or less effective depending on a patient’s genetic makeup; some people, for instance, respond well to the breast-cancer medication taxol, while others may have no response at all. If scientists knew that specific medications worked for certain people or, conversely, caused them to suffer severe side effects, they could use their cells to try to figure out why–and use that information to develop better therapies.
So far, Harvard Stem Cell Institute scientists and their colleagues have used iPS-cell technology to create more than 20 disease-specific stem-cell lines designed to help them study conditions including Parkinson’s and type 1 diabetes. While the field is still in its early stages, researchers have begun to see evidence that they can replicate certain aspects of human disease in a dish.
The first goal for iZumi is to establish its own bank of reprogrammed cells. To start, the bank will be stocked with cells derived from patients with various neurodegenerative diseases–ALS, spinal muscular atrophy, and Parkinson’s–as well as a cardiovascular disorder known as calcific aortic valve disease, which they’re studying in conjunction with collaborators at the Gladstone Institute at the University of California, San Francisco. By creating complex systems of cells that incorporate the different cell types affected in each disease, such as motor neurons and skeletal muscle cells, they can watch precisely how ALS and the other conditions develop.
The company wants to develop drugs, focusing on therapies for neurodegenerative diseases. It will also work with other pharmaceutical companies to find treatments for other diseases. “We believe that we’ll have our own proprietary therapeutics in development in the fifth year–by 2012,” says CEO John Walker.
A Bumpy Road
If iPS-cell scientists have learned anything from the saga of embryonic-stem-cell research, it’s that potential doesn’t always translate into profit or success: despite the vast promise of embryonic stem cells, building a business model around their therapeutic use has been a challenge. Some of the blame can be laid on President George W. Bush. In 2001–citing ethical objections to the process used to obtain the cells, which destroys a days-old embryo–he restricted federal research funding for the technology to a small number of stem-cell lines already in existence. The controversy, the lack of federal investment, and some uncertainty surrounding the science itself made some researchers reluctant to study embryonic stem cells, and many venture capitalists were hesitant to back efforts to commercialize them.
Barack Obama ordered the limits on federal funding removed early in his presidency, but his predecessor’s policies probably set the field back many years. And embryonic stem cells are so finicky and unpredictable that developing treatments based on them has been difficult even apart from the funding obstacles. Only this year, more than a decade after human embryonic stem cells were first isolated, will they finally make it into clinical trials. The first therapy, a treatment for acute spinal-cord injury developed by biotech startup Geron, is headed for trials later this year.
“It’s kind of a ‘good news, bad news’ scenario,” says Daniel Omstead, CEO of Hambrecht and Quist Capital Management. “Every quarter or year, you see new developments that make you very excited about the future but more circumspect about … being able to make money in the near term for investment in technology that will cure disease.” He’s not yet sure whether iPS-cell technology will prove to be the stem-cell field’s home run, and neither are his fellow venture capitalists. “I think many companies will come out of the stem-cell area, but I don’t know that they’ll be focused on iPS cells necessarily,” says Amir Nashat of Polaris Venture Partners, which has funded a company partly based on the technology (see table above).
Stem cells might be easier to commercialize as tools for drug development, an area in which the new technology seems especially promising. But iPS cells still hold many unknowns: they are not as well studied as embryonic stem cells, and there is not yet any standard by which they can be measured. That is one reason no one is yet willing to claim that iPS cells will make embryonic stem cells obsolete; indeed, the inconsistency of iPS cells is one of the biggest research hang-ups at the moment. Researchers don’t quite understand why, but even cells from the same batch can behave very differently. Some are easy to turn into other tissues; some are stubborn. And the rapidly growing repertoire of methods for making iPS cells is adding to the variability.
Only a year ago, researchers had to use a virus to insert the four proteins required to turn an adult cell into an iPS cell. The virus also inserted little bits of itself into the cell’s genome, an invasion that not only prevents therapeutic use but makes lab studies much less reliable. Newer methods use proteins or chemicals, while some techniques still use viruses. Before they can use the cells generated in all these different ways, scientists need to study and document their characteristics. “We just finished initial characterization of a group of 12 lines we made. And then we made some more,” says Jeanne Loring, director of the Center of Regenerative Medicine at Scripps. “So we’re suffering from the same thing everyone else is.” In other words: “Oh my God, we have more lines than we know what to do with.”
But Harvard’s Melton, for one, thinks these problems are only temporary. “This is all solvable in the short term–in the next year or so,” he says. After that, the trick will be figuring out how to prompt the cells to differentiate in the desired ways. There are more than 200 different kinds of cells in the body, and although iPS cells have the potential to turn into any of them, actually getting them to do so is a different story. “How do you tell a cell to become a pancreatic beta cell? How do you tell it to become a four-grain basal cell or a motor neuron?” he says. Scientists have already figured out how to make some neurons and blood cells, to name a few. But they cannot yet efficiently make such important types as pancreatic beta cells, the insulin producers that are destroyed in diabetes. Still, says Melton, “we’re getting closer.”
Though it seems a long way off, scientists still hold out the possibility that iPS-cell technology could one day be used for treatment. “The near-term value for iPS cells is in disease modeling, pathway identification, and drug screening and development,” says George Daley, a stem-cell biologist at Harvard University and Children’s Hospital in Boston. “But I don’t give up hope that we will generate cells that will have therapeutic relevance.”
For now, though, iZumi and other companies are focusing sharply on what they think will be the most immediate use of iPS cells: as tools for understanding some of our most devastating diseases and finding better ways to treat them. The new technology, they hope, will fundamentally change the repetitive, variations-on-a-theme approach to drug development that has hindered pharmaceutical progress in recent years. The discoveries it makes possible could one day transform medicine into something we’re only just beginning to imagine.
Lauren Gravitz is a freelance writer based in Los Angeles, CA.